IC Design: Trajectories from the Past and into the Future
September 23, 2020
Story
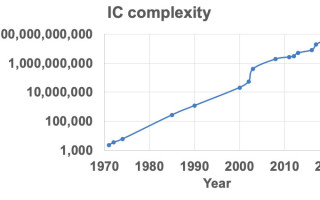
What is the future of IC design jobs? Will AI replace IC designers? These are questions that have been repeatedly asked at many technical conferences I have attended lately.
What is the future of IC design jobs? Will AI replace IC designers? These are questions that have been repeatedly asked at many technical conferences I have attended lately. At the base of every effective AI algorithm is an electronic chip that is designed by a team of intelligent IC designers. Ironically, IC designers could be designing the very technology that may potentially replace their jobs. This, if true, is an intimidating prospect. Yet, by examining the past and present of IC design, we can recognize that IC design today is not what it was in the past. By conjuncture, we can also realize IC design jobs are unlikely to go away. However, they will absolutely shift and become more involved.
Automation, Inefficiency, and Demand
To begin with, there are three patterns in the industry to recognize: automation, inefficiency, and demand. Firstly, present-day IC designers are working with a huge amount of automation technology. The simulation capacity of today’s machines is inherited directly from yesterday’s competitive IC solutions. That is to say, IC design jobs of the past are now automated. The trend towards automation enabled IC designers to move upward in the hierarchy of synthesis. We are now able to make remarkably complex solutions, because we are utilizing innovative, automation technology available to us.
Secondly, there are many IC inefficiencies that remain unsolved. For instance, in RF design, communications are not full duplex yet and antennae are still off chip. If this technology evolved, communications would be double the speed and RF modules would be smaller. Technical issues like these still require continual innovation and focus.
Thirdly, the combination of automation and inefficiency patterns creates a huge demand for clever IC designers to address unresolved problems. We already know how to design a 20dBm PA, so why not let machines do this task for us? Automating processes leaves more time and resources for design teams to troubleshoot new problems. The demand for designers will remain steady until there are no remaining RF and IC inefficiencies. Furthermore, as automation picks up, IC designers in the field will have more fun brainstorming solutions as labor-intensive aspects of the job are eliminated. By nature, IC design jobs are constantly being redefined as breakthroughs occur, and this pivoting will carry into the future as well.
Learning from our past
In order to understand the evolution of design, I interviewed two of our senior IC designers at Silicon Labs. We touched on their earliest memories of IC design and discussed their vision of the future of the industry using only recollection and opinions instead of relying on accompanying documentation.
The first designer I spoke with is a fellow at Silicon Labs. One of the first insights he shared was how the first circuit he designed was a differential Op-Amp and a 20th order switched capacitor filter. The entire analog chip was roughly 30 mm2 and in the order of 5,000 transistors. Comparatively, today’s chips carry tens of Op-Amps and filters and the transistor count can be in the hundreds of millions and above. We have certainly moved towards more complex designs in only a matter of decades. My colleague also described the technology available to designers when he first started his career saying how the layout was done on a Tektronix graphic terminal with a green screen, which is essentially a large storage scope. The terminal did not have colors, but designers worked with one layer of metal and one poly used for routing. Today, designers have access to numerous layers for routing to create devices. Using a colorless monitor in layout nowadays sounds like a project suicide attempt!
(Figure 1. Tektronix 4010 terminal was commonly used for IC layout in the mid-1980s. Source: https://en.wikipedia.org/wiki/Tektronix_4010)
I heard similar sentiments from another engineering fellow who elaborated on his experience with the Tektronix monitor explaining how the monitor consumed about 1kW, was about 4’ tall, had a green screen with persistent graphics, and 2 thumbwheels for X-Y input. The thumbwheel arrangement worked very well and the monitors were driven by a 140kbps cable, which was taped to the floor, across the hall from a computer center room. He also told me designers used to type their netlists on a text editor. They would start with a white board design, number the nodes and then type in a netlist for simulation. Currently, we can only design System on a chip (SoC) ICs, because we have graphic user interfaces that allow us to place and examine thousands of transistors in the analog domain without worrying about node numbers or netlist typos. In this sense, we are leveraging automation to create more complex and advanced systems.
(Table 1 Comparison of circuit complexity between 1980s and today.)
Another old and interesting IC tradition from the mid-1980s was at the chip level. Since designers didn’t have Layout Versus Schematic Software (LVS), they would have to craft together paper printouts of a reasonable scale to create nets. This paper simulation consisted of two or three printouts about 3’ wide taped together on the floor or multiple tables to cover the width of the respective chip. From a top-level netlist, they would verify connectivity to each pin of each block net by net, and then mark the “light up” of each net with colored pencils. While this may seem like an elegant solution given the technology at the time, it was unnecessarily repetitive and tiresome.
(Figure 2. Exponential growth in IC complexity is only allowed by more automation in the design process. Source: https://en.wikipedia.org/wiki/Transistor_count)
Interestingly enough, automation and AI are not new to IC design and rooted in the past. As we have seen, IC designers have always counted on automation to make processes less task-intensive, but now the systems are more intricate than they’ve ever been. However, there are certain processes that are still solely done by human minds, like analog design. Analog design is the intuitive process of sizing devices and achieving analog functions using specific device configuration. While computers help with math and estimate operating points of circuits, human minds are better at intuitive, intelligent design. Now, the question is whether recent developments in AI make machines intelligent enough to replace IC designers?
From here, we can speculate a few outcomes. First, automation of such complex design principles should be viewed as a good thing, and machines should be viewed as an extension of the human mind. As computers get smarter, humans have more time to focus on novel concepts and reach new milestones. Furthermore, automating the design process has been happening for decades already. In the 1980s, one of my long-time designer colleagues explained how a few engineers at Bell Labs successfully automated design of Op-Amps and switched capacitor filters. They essentially used known topologies and then used optimization methods to choose component sizes. As an IC designer, I find this automation comforting as I enjoy the critical thinking behind choosing a topology more than the laborious aspects of optimizing components.
Human vs. Machine: How reliable are machines?
The stories I learned about the 1980s from my colleagues demonstrate that human intelligence in IC design is truly needed. For example, one of the stories described how an in-house group of math specialists at Bell Labs undertook a project about applying constraints and minimizing the number of simulation trails required to do an optimization. To do this, they handed off a band-gap design with the goal of minimizing percentage change in output voltage across PVT and mismatch, while keeping PSRR and power below some bound. They decided to simplify the optimized parameter to mV change instead of percentage change. After several weeks, they returned with a presentation and were proud that they had reduced the variability by orders of magnitude, which initially seemed too good to be true. Surprisingly, their tools had discovered a resistor that could be sized down to near zero to reduce the output voltage to just a few mV with a very small variability (measured in mV) across PVT!
Should we ascribe the outcome of this incident to machines or humans? Machines made by humans will err as much as humans simply due to the fact that they are made by us. This is why a human observer will always be needed at the top of any automated process. For instance, modern-day computing speed is exponentially higher, which allows us to run every possible variation of a chip to confer whether it will work or not. While speed is great, incorporating a real human in this process guarantees a higher probability that a problem will be detected —especially in an analog system. Thus, I truly believe we cannot rely on machines solely to detect problems since problems can perpetuate in the machine. We must accept that humans are imperfect, and machines will be imperfect by nature.
The stories I picked from the past highlight the contrast between humans versus machines. However, similar situations continue to occur as researchers and entrepreneurs’ experiment more with automation of design processes. Compared to the past though, I speculate the evolution of future automation will focus on tougher problems that might not even be on our horizon yet.
Originally, engineers built simpler systems but took on all the burden of analysis. Coming up with new designs and building analytical models requires enormous mental effort. Without access to simulators and other automation processes, engineering capacity was limited to thousands of transistors. Luckily, as technology advances, we can test ideas in a matter of hours without establishing analytical models. For example, there is more simulation bandwidth to verify functionality of billion transistor SoCs.
On the other hand, as complexity increases and chips become more massive, the validation and verification cycles also get longer, and consequently, more laborious. As one of my colleagues likes to say, we spend 10% of our time coming up with clever ideas, but 90% of our time simply verifying said ideas.
Evolution: what can we expect in the job market?
Looking at the trajectory from past to present establishes an extrapolation towards the future with an exciting possibility. More automation opens up time for creative and critical thinking. Perhaps very soon, machines will be able to replicate designs, or even suggest new design ideas. Machines will also take part in the tedious verification process. This will afford IC designers the luxury of working on “fun and exciting problems.” While these visions are ideal, we shouldn’t be too eager for AI to completely take over in the near future. As a first step, AI could start automating floor planning, and then layout. Then, AI could slowly crawl towards the core design functions, creating more mental space for designers to focus on new problems.
(Figure 3. ICs get more complex. Designers go back to creative tasks and drop laborious processes to AI.)
In terms of IC design jobs, teamwork and coordination will become more relevant. Design teams are growing to cope with the humongous scale of modern ICs. Communication between engineers and engineering functions might occupy more bandwidth. If we want to build the massive systems that we only dream about today, future IC designers will need to be collaborative decision makers. Addressing collaboration will be an educational and cultural issue as well. Instead of just technical skills, STEM students need to focus on soft and interpersonal skills like communication, creativity, imagination and teamwork. The human mind is capable of powerful things when it is stimulated in all areas and working with other great minds.
A young engineering student once asked me if she should turn the page and shift from hardware engineering to software development. A common concern among young engineers is whether their field of study is needed in the market or not. My response to this dilemma is to always encourage students to learn about the market themselves and see how to position themselves for success. I can’t speak to which job is better or which job pays more since passion determines career satisfaction. This journey is very personal. Speaking from my experience only, I can assure students who are curious about IC design that the demand for her skillset won’t go away anytime soon, as there are many problems to solve. The difference is that the types of problems in the future may be radically different due to the disruption of AI and automation. This is anyone’s game to predict. However, I am very hopeful that we are entering a future where humans and machines can harmoniously work together.
For curious readers, here are some papers on automation from the 1980s to examine:
• Bell Labs ~1985
– Fatehy el-Turky
• Opamp design: optimization of in-house opamp topologies
• “BLADES: An Artificial Intelligence Approach to Analog Design,” IEEE Trans. CAD June 1989
– Toshio Misawa
• Automated design of switched-capacitor filters
– Cascade of Fleischer-Laker biquads
• Publications (small sample):
– P. Allen, et al, “AIDE2: An Automated Analog IC Design System,” CICC 1985
– R. Harjani, et. Al., “A Prototype Framework for Knowledge-Based Analog Circuit Synthesis,” IEEE DAC, 1987.
– H. Koh, et. Al, “Automatic Synthesis of Operational Amplifiers Based on Analytic Circuit Models,” ICCAD, 1987.
– A. Barlow et.al.,”An Integrated Switched-Capacitor Filter Design System,” CICC 1989
About the Interview Subject
Special thanks to interview subjects and Silicon Labs Fellows Dr. John Khoury and Jeff L. Sonntag for the development of this article. Dr. Khoury served as an engineering professor at Columbia University and held previous engineering and management positions at Bell Labs and Multilink Technology. He earned engineering degrees at Columbia and MIT. Jeff Sonntag has engineering degrees from Cornell University and Carnegie Mellon University. Prior to Silicon Labs, he spent 18 years at Bell Labs where he was recognized as a Bell Labs Fellow for his contributions.
About the Author
Asem Elshimi is an RFIC design engineer for IoT wireless solutions at Silicon Labs. He joined Silicon Labs in July 2018. Elshimi specializes in the areas of RF circuit design and electromagnetic structure design. He holds an M.S. in Electrical and Computer Engineering from the University of California, Davis.