Harvesting nanowatts to milliwatts from ambient sources using advanced energy harvester integrated circuits
March 14, 2016
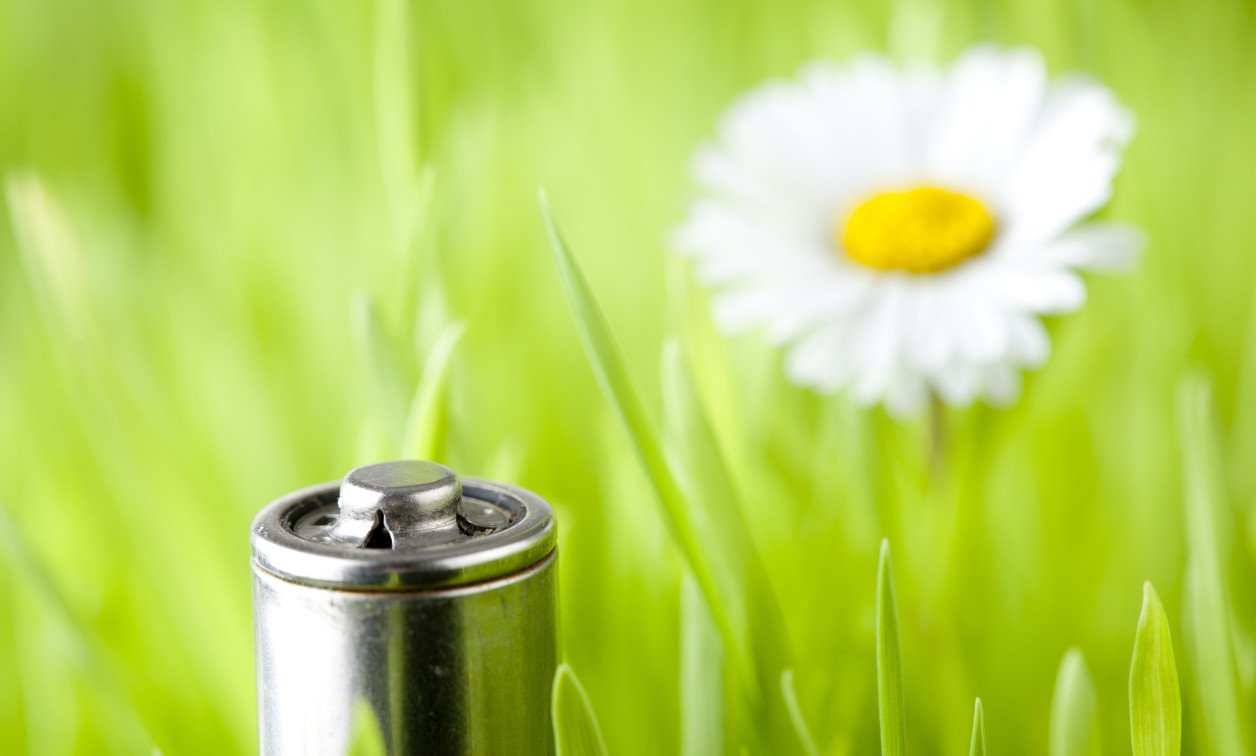
In the context of energy harvesting, advanced power management integrated circuits (PMICs) function like a utility company in that it controls the del...
In the context of energy harvesting, advanced power management integrated circuits (PMICs) function like a utility company in that it controls the delivery of ambient power to downstream electronics. Properly selecting a PMIC based on five important criteria can allow system designers to augment system batteries or create self-powered systems.
An energy harvester is an electrical power source. It operates by absorbing energy present in the ambient source and then presenting this absorbed energy at its output to be used by downstream electronics. Some common examples of harvesters are silicon devices that exhibit the photovoltaic effect (solar cells) and the thermoelectric effect (thermoelectric generators). The former acts as a power source by converting impinging light energy to create an electrical voltage; the latter converts temperature differences (thermal energy) to an electrical voltage.
In both cases, electric current flows when the source is connected to an electrical load, and thus power is transferred from the ambient source to the device under operation. Solar cells and thermal electric generators (TEGs) can be likened to a battery. Both have no moving parts and can provide power to run electronics. Some harvesters, however, like the 1,000-year-old windmill or its modern version – the wind-turbine – do have moving parts. Others that are based on the piezoelectric or electromagnetic effect also have moving parts. Piezoelectric harvesters are silicon-based devices that harvest motion; they convert kinetic energy to electrical energy.
While the harvester is an important piece of the energy harvesting puzzle, in most cases it cannot function alone. Another piece of silicon, the power-conditioning IC, is vitally important. This power-conditioning IC, also known as the energy harvesting PMIC, is the critical link between the harvester itself and the downstream electronics. The PMIC functions like the gatekeeper or the utility company in that it controls the delivery of ambient power to the user.
The energy harvesting PMIC
Unlike a battery that provides power for a limited time, a harvester can provide power for eternity – or at least for a very long time, which can measure in years or even decades. One drawback of many harvesters is that they are a very weak or low-energy source. A weak energy source will not be able to satisfy the demands of today’s power-hungry applications. Some harvesters provide energy intermittently (most solar cells will not operate under low-light conditions, such as in darkness). The role of the PMIC is to receive weak and intermittent energy, and to condition and transform this energy into a usable form.
An effective energy-harvesting IC should be able to perform the following important functions:
- Provide the necessary electrical interface to the harvester to ensure maximum power transfer under the given ambient conditions.
- Receive the energy output by the harvester when conditions are good and store this energy in a storage device for later use.
- Manage (control) the power transfer to the electrical load (typically downstream electronics) while making sure that high energy demands from the load do not expose the system to the risk of abrupt power loss and the resultant system failure.
- Be able to manage its own start-up using only the weak harvester source in case the rest of the system is dead due to loss of power from the storage device.
- Use very little energy to keep itself running, in other words, maximize energy transfer from the harvester source to the storage element. This is especially critical when the source is very weak.
Now let’s review the important aspects of each of these five functions.
Maximum power point tracking
Harvesters like the solar cell and a TEG have a complex relationship between their excitation source (light and temperature, respectively), and the power they produce. For any given set of external conditions, they have a single operating point that produces maximum power. It is the job of the energy-harvesting PMIC to “present” and “track” a load resistance to the harvester that forces it to operate in its maximum power point region, depending on the ambient conditions. As the ambient conditions change (for example it gets brighter or darker), an energy harvesting PMIC with the maximum power point tracking (MPPT) feature will be able to adjust such that maximum power is drawn under all conditions.
There are several strategies to create an effective MPPT scheme. The most commonly used for its simplicity and relatively high accuracy is the open circuit voltage scheme (VOC). In this method, the output voltage of the harvester is regulated to a fixed value, which is an empirically derived ratio of the periodically measured “open circuit voltage” (under zero load current conditions). For example, most solar cells can be regulated to between 75 and 80 percent of their VOC to operate in their maximum power point region.
In our example using the bq25570 energy-harvesting PMIC, the MPPT can be easily implemented using an external resistor network (Figure 1).
Energy storage management
The energy-harvesting PMIC performs the critical role of receiving energy from the harvester and storing it in a storage element for later on-demand use. Most energy-harvesting PMICs work with a wide variety of storage elements, such as conventional capacitors, supercapacitors, Lithium-Ion (Li-Ion) rechargeable batteries, and thin-film batteries.
As most storage elements have an upper limit of charge and a lower limit of discharge, accurate management of these and other related parameters is very critical in an energy-harvesting system. Features such as an indication that battery level (or storage element) is “good” or “not good” are important in designing a flexible system. Many energy harvesting PMICs provide these aforementioned functions, offering system designers the flexibility to set external resistors in order to manage the charging and discharging levels of the storage element, as well as the “battery good” level setting (Figure 2).
Managing power transfer to the electrical load
For safe operation of the system, the “health” of the storage element is used to determine when a load can be applied or should be removed. Typically, energy-harvesting PMICs will monitor the voltage on the storage element to provide an indication (signal) of whether the storage element is good.
As long as the voltage is not below a pre-determined value, the load can be applied and will be allowed to consume power. If the voltage of the storage element falls below that pre-determined value, the PMIC can provide a signal to the microprocessor and take some preventative actions. For example, the duty cycle may be reduced, or only certain “keep alive” circuits will be allowed to be powered to conserve battery life.
In the system depicted in Figure 2, this can be achieved by using a switch between the regulated output pin and the load. The switch can be opened or closed by the microprocessor based on the status of the VBAT_OK signal.
Manage its own power-up – The cold startup
The cold startup circuitry is vital for system operation when the storage element is fully depleted and the system has no indigenous power. In such cases, the energy management PMIC has to rely on the harvester to power up. However, as mentioned, harvesters can be weak energy sources, outputting only a few hundred millivolts, so it follows that the energy harvesting PMIC must be able to startup using the few hundred millivolts from the harvester. With the wide variety of energy harvester ICs available, each have their own specific cold startup specification. For instance, the bq25570 startup occurs at approximately 330 mV.
Quiescent current – The silent killer
Quiescent current of the energy harvester PMIC needs be as low as possible. Keeping this current low minimizes the overall system-sleep current. An energy harvesting system usually spends most of its life sleeping, wakes up intermittently to make and transmit measurements, then goes back to sleep again. The more time spent in sleep, the more sleep current becomes paramount to battery life. Arguably, no other system parameter has a greater impact on the success or failure of an energy harvesting system than sleep current. When it comes to quiescent current, not all energy-harvesting PMICs are born equal. The range of available options varies dramatically. Some have quiescent currents in the milliamperes, while a select few have quiescent currents in nano-amperes, the latter being the best of the best.
Summary
The energy harvesting PMIC is a critical component of an ultra-low-power energy harvesting system that operates in the nanowatt to milliwatt range. Such systems are finding a wide range of applications, such as sensors for industrial as well as consumer. Often the sensors are battery-operated, and extending the battery life is a big concern for system designers.
There are many factors to consider when selecting an energy harvesting IC. In this article we examined some of the more important features of these integrated circuits – such as maximum power point tracking, cold startup, energy storage, managing the load, and quiescent current. Properly selecting an energy harvesting IC allows system designers to creatively use ambient energy to self-power their applications, or augment the on-board battery to provide longer run time.
Texas Instruments
www.ti.com
References
Pathare, Niranjan. Energy harvesting is possible on Mars, Fully Charged, TI blog, January 8, 2016.
What do oil rigs, fitness equipment, and cars have in common? TI’s latest innovation in energy harvesting, Think. Innovate, TI blog, November 19, 2013.