Edge Computing Innovation: Doing More with Less
September 03, 2020
Story
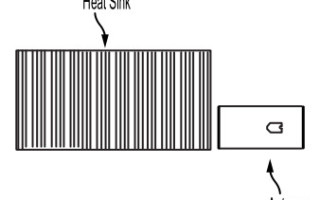
The pandemic has essentially accelerated the need and reliance on edge computing, which offers vast potential for supporting not only today's demands, but also the demands of future applications.
The COVID-19 pandemic is transforming how we are using data, and where that data is consumed and generated. The data usage model has shifted from a more traditional, centralized one, to one that must support many remote users and connection points. These remote connections are quickly changing the existing telecom architecture, fostering the need and development of a distributed architecture where the edge devices now define the access points and their functions.
The pandemic has driven greater demand of networking and computing in support of some key activities, including:
- Remote working – at home or in remote offices/ branch offices (ROBO)
- Remote learning
- Remote healthcare (telemedicine)
These drivers build upon an ever-increasing demand for media and entertainment (M&E) applications such as video streaming and gaming. In addition, the telecommunication industry has been continuously improving urban and suburban last-mile connectivity, with today’s emphasis on expanding wireless 5G connectivity. This will support a huge potential increase in data creation.
Today, network-based processing is used extensively to support smart watch apps, streaming video, smart home applications, and much more. However, much of this processing relies on a centralized architectural approach, in which all of the collected data must be backhauled to a central “cloud” datacenter, where it is processed. These processing results are then converted to specific actions that may require it to be taken back at the data source. The cost of moving all of that data, and the delay incurred as a result of this approach, does not readily support real-time applications, such as Augmented Reality, conversational intelligence, or autonomous systems (self-driving cars/trucks/ships, robotic factories, and the like). Content-rich applications demanding low latency and high throughput require a combination of access (connectivity) and compute resources at the edge of the network.
Edge computing and its distributed processing model is designed to meet this need. This approach places computation, storage, and control services closer to the billions of “Internet of Things” devices like sensors, actuators, cameras, and of course, users and their devices. Successful implementations will place computational resources at optimal points in the communication infrastructure, whether in the central cloud, the edge compute nodes, or the end devices themselves. And it will be able to dynamically scale resources to meet the changing needs at these different points.
The pandemic has essentially accelerated the need and reliance on edge computing, which offers vast potential for supporting not only today’s demands, but also the demands of future applications that require localized processing for rapid response and core bandwidth reduction. The shifts in access location and content delivery at the edge of the network was not expected to occur so quickly, and it is now forcing the industry to alter how it enables and delivers content while at the same time providing a secure environment from which to deliver and use the content.
Performing complex data computation locally - proximity processing - is now crucial. And it can be made significantly more powerful if these edge computing resources were placed within 30 meters of the endpoints. This, however, requires further technical innovation.
Edge computing devices utilize both wired and wireless connectivity methods. The wired connectivity method used is predominantly ethernet. However, most of the methods used for providing connectivity to and from the edge device utilize wireless communication for radio access.
Unfortunately, there is no single network or radio access method which can solve all the connectivity and data challenges. For example, some of the basic wireless connectivity protocols include LTE, 5G wireless, WiFi (802.11ax and 6e), Bluetooth, BLE, ZigBee, and others. Therefore, this new class of edge computing devices should support multiple radio access methods. However, the large number of wireless radio protocols competing for real estate and power on the edge computing device is driving the need for increasing levels of functional integration.
To better support low-latency, high-bandwidth applications, powerful, integrated consumer-facing edge computing gateways, which we refer to as Smart Edge Nodes, or SENs, are beginning to emerge. These SENs support multiple radio access methods along with increased amounts of secure computing, storage, and routing capabilities. They are built with more powerful processors, systems-on-chip (SoCs) devices, memories, antennas, power amplifiers, and other resources to better support high-speed wireless communications while simultaneously executing complex, power intensive applications at the device-edge of the network.
That brings us to heat. As functions and capabilities are added to any device, the amount of power and associated heat increases. This heat must be removed, or the electronics will stop working reliably. One typical method used to dissipate this heat is to simply increase the physical size of the device to increase the power-dissipating surface area. However, simply increasing the physical size is often counter to the desired goal of maintaining or even reducing a SEN’s physical size as capabilities are added. The market always wants us to provide more - with less. In this case, less horizontal and vertical surface area is available for heat dissipation. In addition, edge devices are often deployed or used in environments that preclude other thermal management solutions, such as forced airflow or external cooling prevalent in cloud datacenters.
Due to these physical constraints, high-power edge-deployed equipment form-factors are often driven by heat dissipation. For example, a SEN can be shaped as a quadrilateral cuboid having two elongated sides or include large casings that house large heat sinks to increase heat dissipating surface area.
Beyond the heat, a SEN for use in homes and small offices must comply with certain size and physical design requirements that do not require a server rack installation. Since the SEN is sharing a physical environment with people, it should be either unobtrusive or physically attractive, so that it may be readily placed throughout a home or small office. At the same time, these physical attributes must not impede performance, functionality, and future-proofing or drive cost.
Many product miniaturization advancements for the benefit of wireless communication have taken place; just look at your smartphone today, compared to the smartphone-brick of the late 20th century. Of course, as more radio access protocols and frequency bands are added to our edge devices, physics demands the need for more antennas. When a new radio technology is added, the additional power mandates a larger heat sink. The easy solution is to increase the size of the platform to accommodate more/larger heat sinks as the number of antennas increases. Unfortunately, this is not what the market is willing to pay for.
The number of antennas and size of the heat sink(s) are key drivers for determining the overall size of the SEN itself. When more radio access capability is added, typical design approaches demand more physical real estate for each new wireless protocol or frequency band to be supported. How do we break this apparent logjam?
What if a heat sink and antenna were one and the same? Recent advancements have successfully integrated antennas with heat sinks, supporting the “do more with less” market requirements. This area of integration presents an opportunity for further innovation.
Figure 1 shows a Planar Inverted F-antenna (PIFA) and heatsink which are used extensively in wireless communications. The conventional design approach dictates that there is a heat sink area and an RF antenna area and the two shall never meet. However, SENs use multiple radios operating in different frequency bands. Many radio systems like Wi-Fi ax and Wi-Fi 6e require multiple frequency bands and antennas to deliver the massive bandwidth required, resulting in an array of radios and antennas.
(Figure 1 Heat Sink and Antenna)
An integration antenna with heatsink is shown in Figure 2. We have found that the integrated heatsink and antenna are symbiotic, operating as joint tenants to the same real estate. Specifically, the heatsink forms a larger ground plane for the antenna, enabling a better performing antenna with the added benefit of a reduced footprint. The antenna also acts as a heatsink helping dissipate heat that is generated. This combined antenna and heatsink enables greater power management and wireless performance in smaller area. When innovation is successfully brought to bear, everyone wins.
(Figure 2 Combined Heatsink and antenna)
With this integrated antenna/heatsink, the SEN can dissipate the same amount of heat and achieve the same thermal performance as a much larger conventional device, while simultaneously meeting radio performance requirements. By having fewer parts, this combined antenna/heatsink combination also reduces material and manufacturing costs which can be used to increase margins or increase market price competitiveness.
We fully expect that the integrated antenna/heatsink innovation will help foster a new era of product miniaturization, not only for the advancement of Smart Edge Nodes for edge computing, but also in many other non-edge computing applications.