Medical Marvels: The Pairing of Wireless Devices and Connectivity
September 26, 2023
Blog
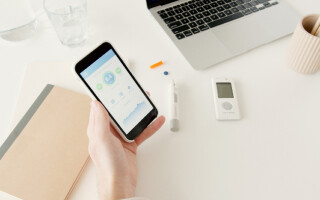
Over the last 50 years, electronic devices have become increasingly important tools to aid medical professionals in the diagnosis and treatment of disease. Early examples include the scanners (CT, MRI) developed in the 1970s which offered breakthroughs in the level of detail that could be seen inside the body.
These early machines were confined to major hospitals, because they were large, expensive, and required complex maintenance. The subsequent development of microelectronics has enabled a highly diverse range of wearable personal medical- or health-related devices. The emergent ubiquitous connectivity allows these wireless devices to communicate with the cloud and/or medical professionals, thus enhancing their value.
Wearable Technology
Simple fitness trackers were one of the first examples of a health-oriented wearable. Whilst they did not claim to have clinical value, from an electronics design perspective, they possessed most of the classic characteristics of a medical wearable.
Such a device is simple in terms of the electronics schematic. A small microprocessor is connected to a variety of sensors, and often a radio, either as a separate device or integrated into the processor, is present to transmit data onwards. The challenge in design lies in the following areas:
- Designing the layout and packaging of the device to make an ergonomically practical device.
- Ensuring the sensors read data reliably and processing this data to give useful information.
- Dealing with the proximity to the human body for devices with a radio from both a safety and reliable data transmission perspective.
- Managing power consumption to make a practical device.
“System in Package” Techniques
Standard electronic components have become progressively smaller over the years. Nevertheless, designing a practical wearable often requires advanced techniques. System in Package (SiP) technology offers one approach whereby semiconductors, in chip scale packages, can be combined with smallest form factor components to condense complex functions into a miniature component. A device may have to be adapted to the part of the body where it is designed to be placed. An obvious example is the wrist, where a PCB might need to be separated into different sections connected by some type of flexible PCB. The Apple Watch, for example, includes several SiP devices, without which it cannot achieve its form factor.
Data Analysis
Much of the value in a medical device is in the treatment of data. Whilst a simple fitness tracker might provide an accurate heart rate, a more advanced device might be able to detect issues in the heart rhythm. It is unlikely that such processing could be conducted on the device itself due to the limited resources available. So, in this case, connectivity is key to transfer data to a more powerful computer. This could be simply to the user’s mobile phone, or in more complex cases from there onward to the cloud.
Whilst simple medical/health devices are products, a more advanced device can be sold as a service, where the wearable hardware is part of a solution involving cloud-based analysis and reporting and monitoring by a medical professional.
Wireless Technology on the Body
All but the simplest devices exploit wireless connectivity. Unfortunately, the human body is not a friendly place for radio transmissions. For the 2.4Ghz band, it acts as a near perfect absorber of radiation. In free space, one can expect a range of maybe 50m for a Bluetooth Low Energy (BLE) signal. However, place it close to the body, and hope to transmit to a phone on the other side of the person, and you might drop to 1m or less.
However, there are several design techniques that can be applied to enhance performance. Starting with a device with strong performance is critical. Placement of the antenna is also crucial. Simulations by Insight SIP have shown even relatively small displacement (mm level) of the antenna from the skin surface can have a dramatic impact on performance. Another aspect to consider is that placement of the antenna close to the body can “detune” the antenna so the peak frequency is displaced. There a truly optimal solution might be to design a custom antenna to match the positioning of the device. However, this is a complex and specialist task.
What matters is if the range/performance is good enough – if the requirements are simply to connect to a phone carried on the person, or in the same room - then intelligent placement of a high performing standard device should be enough.
Safety Issues
One must also consider regulations on wireless transmission close to the body, known as SAR (Specific Absorption of Radiation). The rules for this are different for different regions. In the European Union, the rule is that there is a maximum of 2W/Kg, averaged over the 10g of tissue with the highest absorption of radiation. For the United States, the rule is more stringent, with a limit of 1.6W/Kg, averaged over 1g of tissue. However, there is an exemption for any device that transmits less than 1mW on average.
This latter exemption is particularly interesting for devices using BLE, as one of the advantages of BLE is that devices go to sleep when not transmitting. So, the “on average” part of the exemption becomes important as BLE devices are typically only transmitting a fraction of the time. At 0dBm, the output power is only 1mW anyway, so there is no issue. At +8dBm, a typical maximum figure for BLE devices, the output power is around 6mW. So, if the device is transmitting less than one-sixth of the time, the SAR exemption will be met. It is hard to imagine any medical device that would require the radio to be anywhere near permanently transmitting, since the data being transmitted is typically acquired from sensors on the device itself. The 2Mbps data rate of BLE is more than sufficient to monitor changes in the human body, given that changes in human vital signs change slowly.
For other protocols, the situation might be different, particularly those that require a permanent connection or operate at higher output power levels.
Power Consumption
Power consumption is an issue for any wireless wearable device. It has been shown that the need for frequent recharging is a major disincentive for adoption of wireless devices. Indeed, this was the original issue with Bluetooth prior to BLE. We might be willing to charge our phones daily, but few people want to charge multiple devices. To optimize power consumption, it is important to follow some basic principles:
- The most power-hungry activity is typically the radio. Thus, minimizing the data to be transmitted, perhaps by pre-processing it, and transmitting efficiently are key.
- Managing the connection efficiently. There will inevitably be times when the other end of the connection is unavailable; repeated attempts to connect to something that is not there will be wasteful.
- Intelligent use of sleep mode. Most systems have low current sleep modes. So, making the most use of these modes when there is no activity is critical.
Future Evolution of Medical Devices
The next step in medical devices could be a significant expansion of wirelessly networked, implantable devices. The venerable pacemaker is an old implantable device, the first one being inserted as far back as 1958. However, this was designed as a standalone device to run independently. More recently connectivity has been added to pacemakers and other implantable devices such as continuous glucose monitoring solutions for diabetic patients.
The issue here is that all the issues above related to proximity to the body, and power consumption, are amplified further when trying to get a signal out of the body from inside. Solutions have tended to require a receiver or relay device to be placed close to the implantable. However, advances in technology – higher sensitivity and lower power devices - are open to the possibility of implantable devices that can communicate directly with a smartphone and provide even richer data. This could lead to the early detection of disease before there are any evident symptoms, by, for example, the monitoring of blood chemistry. With parallel developments in Artificial intelligence (AI), one can easily imagine a situation where potential health issues could be diagnosed without human intervention.
Conclusion
Health care services are under pressure across the world driven by increasing demand for advanced treatments and in many countries, an aging population with increasing health needs.
Wireless health technology can play a key role in meeting this challenge, by providing closer “real-time” monitoring of health conditions, and early detection of disease. It has repeatedly been shown that early diagnosis can save a factor of ten or more in treatment costs. Connected devices linked to analysis software in the cloud and (if necessary) medical professionals can short circuit a long appointment/test/results cycle.
They can also allow users to take better control of their own health and fitness, by providing quick feedback in an easy to access form on their familiar mobile devices.
The design of ergonomic and high performance wireless body worn devices can be a challenge, but ever improving technology coupled with creative ideas will undoubtedly mean this sector is poised for robust growth going forward.