Reduce Power Supply Requirements for Ceramic Capacitors in Industrial and Automotive Applications
June 04, 2020
Story
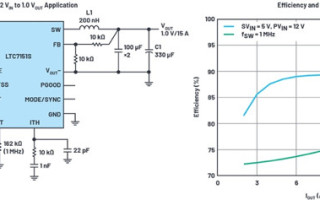
The price of the multilayer ceramic capacitors (MLCCs) has risen sharply over the past several years, tracking the expansion in the number of power supplies used in various industries.
The price of the multilayer ceramic capacitors (MLCCs) has risen sharply over the past several years, tracking the expansion in the number of power supplies used in the automotive, industry, data center, and telecommunications industries. Ceramic capacitors are used in power supplies on the output to lower output ripple, and to control output voltage overshot and undershot due to high slew rate load transients. The input side requires ceramic capacitors for decoupling and to filter EMI due to their low ESR and low ESL in high frequency.
The quest to increase the performance of industrial and automotive systems calls for increases in data processing speed of several orders of magnitude, with an increasing number of power-hungry devices squeezed into microprocessors, CPUs, system on chips (SoCs), ASICs, and FPGAs. Each of these complex device types requires a number of regulated voltage rails: typically, 0.8 V for cores, 1.2 V and 1.1 V for DDR3 and LPDDR4, respectively, and 5 V, 3.3 V, and 1.8 V for peripheral and auxiliary components. Buck (step-down) converters are widely used to produce the regulated power supplies from a battery or a dc bus.
For instance, the proliferation of the advanced driver assistance systems (ADAS) in automobiles has dramatically increased ceramic capacitor usage rates. With the rise of 5G technology in telecommunications, where high performance power supplies are required, ceramic capacitor usages will also significantly increase. Core supply currents have increased from several amps to tens of amps, with very tight control of supply ripple, load transient overshot/undershot, and electromagnetic interference (EMI)—features that require additional capacitance.
In many cases, traditional power supply approaches can’t keep up with the pace of change. Overall solution size is too big, efficiency is too low, circuit design is too complicated, and the bill of materials (BOM) is too costly. For example, to meet the tight voltage regulation specifications for a fast load transient, a large number of ceramic capacitors are required at the output to store and source significant currents arising from load transients. The total cost of the output ceramic capacitors can reach several times that of the power IC.
Higher power supply operating (switching) frequencies can reduce the effect of transients on output voltage and reduce the capacitance requirements and overall solution size, but higher switching frequencies usually result in increased switching losses, reducing overall efficiency. Is it possible to avoid this trade-off and meet transient requirements at the very high current levels demanded by advanced microprocessors, CPUs, SoCs, ASICs, and FPGAs? To consider this question, let’s look at a 20 V input to 1 V at 15 A output for an SoC.
15 A from 20 V Input
Figure 1 shows a 1 MHz, 1.0 V, 15 A architecture for SoC and CPU power applications where the input is typically 12 V or 5 V and can vary from 3.1 V to 20 V. Only input and output capacitors, an inductor, and several small resistors and capacitors are necessary to complete a power supply. This circuit can be easily modified to produce other output voltages, such as 1.8 V, 1.1 V, and 0.85 V, down to 0.6 V. The negative return (to the V– pin) of the output rail enables remote feedback sensing of the output voltage close to the load, minimizing feedback errors caused by voltage drops across board traces.
The approach shown in Figure 1 uses a regulator with high performance integrated MOSFETs. This particular regulator is a LTC7151S monolithic buck regulator which uses a Silent Switcher 2 architecture to simplify EMI filter design. Available in a 28-lead, thermally enhanced 4 mm × 5 mm × 0.74 mm LQFN package. Control is via valley current mode and reduces output capacitance requirements. Protection functions are built-in to minimize the number of external protection components.
The minimum on time of the top switch is only 20 ns (typical), enabling direct step-down to core voltages at a very high frequency. Thermal management features enable reliable and continuous delivery current up to 15 A from input voltage up to 20 V without heatsink or airflow, making it a popular choice for SOCs, FPGAs, DSPs, GPUs, and microprocessors in telecom, industry, transportation, and automotive applications. With a wide input range, the regulator can be used as a first-stage intermediate converter, supporting up to 15 A at 5 V or 3.3 V to multiple downstream point-of-load or LDO regulators.
Figure 1. Schematic and efficiency of a 1 MHz, 15 A buck regulator for SoCs and CPUs.
Meet Tight Transient Specifications with a Minimum Output Capacitor
Typically, an output capacitor is scaled to meet requirements for loop stability and load transient response. These specifications are particularly tight for power supplies that serve processor core voltages, where load transient overshoot and undershoot must be well controlled. For instance, during a load step, the output capacitor must step in, instantaneously supplying current to support the load, until the feedback loop brings up the switch current enough to take over. Typically, overshoot and undershoot are suppressed by installing a significant number of multilayer ceramic capacitors at the output side, fulfilling charge storage requirements during fast load transients.
Additionally, pushing the switching frequency higher can improve fast loop response, but at the price of increased switching losses.
There is a third option: regulators with valley current-mode control can dynamically change the regulator’s switch TON and TOFF times to almost instantaneously meet the demands of load transients. This allows for significantly reduced out-put capacitance to meet fast response times. Figure 2 shows the results of the LTC7151S Silent Switcher regulator immediately responding to a load step from 4 A to 12 A with 8 A/µs slew rate. The controlled on-time (COT) valley current-mode architecture of the LTC7151S allows the switch node pulses to compress during the 4 A to 12 A load step transition. About 1 µs after the start of the rising edge, the output voltage starts its recovery, with overshoot and undershoot limited to 46 mV peak-to-peak. The three 100 µF ceramic capacitors shown in Figure 2a are sufficient to meet typical transient specifications, as shown in Figure 2b. Figure 2c shows typical switch waveforms during a load step.
Figure 2. (a) This 5 V input to 1 V output application runs at 2 MHz, requiring minimal capacitance at the output to quickly and cleanly react to (b) load steps, along with (c) switching waveforms during the load step.
High Efficiency Step-Down at 3 MHz Fits Tight Spaces
Using a high integrated regulator keeps MOSFETs, drivers, and hot-loop capacitors close. This reduces parasitic effects and allows for fast turn on/off of the switches with very narrow deadtime. The conduction loss of the antiparallel diode of the switches is greatly reduced. The integrated hot-loop decoupling capacitor and built-in compensation circuit also eliminate design complexity, minimizing the total solution size.
As previously mentioned, the 20 ns (typical) minimum on the top switch allows for very low duty ratio conversion at high frequency, enabling the designer to take advantage of very high frequency operation (such as 3 MHz) to reduce the size and value of the inductor, input capacitor, and output capacitor. Extremely compact solutions are possible for limited space applications, such as portable devices or instruments in automotive and medical applications. Bulky thermal mitigation components such as fans and heatsinks are not necessary when using the LTC7151S, thanks to its high-performance power conversion, even at very high frequencies.
Figure 3 shows a 5 V to 1 V solution operating at a 3 MHz switching frequency. A small size 100 nH inductor from Eaton together with three 100 µF/1210 ceramic capacitors delivers a very low-profile compact solution for FPGA and microprocessor applications. The efficiency curve is shown in Figure 3b. The temperature rise at room temperature is about 15°C at full load.
Figure 3. Schematic and efficiency for 5 V input to 1 V/15 A with fSW = 3 MHz.
Improving EMI Performance
Meeting published EMI specifications, such as CISPR 22/CISPR 32 conducted and radiated EMI peak limits, with a 15 A application could mean a number of iterative board spins, involving numerous trade-offs of solution size, total efficiency, reliability, and complexity. Traditional approaches control the EMI by slowing down switching edges and/or lowering the switching frequency. Both have undesired effects, such as reduced efficiency, increased minimum on and off times, and a larger solution size. Brute force EMI mitigation, such as complicated and bulky EMI filters or metal shielding, add significant costs in required board space, components, and assembly, while complicating thermal management and testing.
EMI can be reduced in a number of ways, including integrated hot-loop capacitors, to minimize noisy antenna size. The LTC7151S keeps EMI low by incorporating high performance MOSFETs and drivers, which allows the IC designer to produce a device with built-in minimized switch-node ringing. The result is that the associated energy stored in the hot loop is highly controlled, even when switching edges have high slew rates, enabling exceptional EMI performance while minimizing the ac switching losses at high operating frequencies.
The LTC7151S has been tested in an EMI testing chamber and passed the CISPR 22/CISPR 32 conducted and radiated EMI peak limit with a simple EMI filter in front. Figure 4 shows the schematic of a 1 MHz, 1.2 V/15 A circuit, and Figure 5 shows the radiated EMI CISPR 22 test result in gigahertz transverse electromagnetic (GTEM) cell.
Figure 4. Schematic of a 1.2 V regulator with 1 MHz switching frequency.
Figure 5. Radiated EMI in GTEM passes the CISPR 22 Class B limit.
The proliferation of intelligent electronics, automation, and sensors in industry and automotive environments has driven up the required number and performance requirements of power supplies. Low EMI, in particular, has increased in priority as a key power supply parametric consideration, along with the usual demands for small solution size, high efficiency, thermal proficiency, robustness, and ease of use. With an integrated regulator, developers can meet stringent EMI demands in a very compact. With valley current-mode control and high frequency operation, regulators can dynamically change TON and TOFF times to actively support load transients nearly instantaneously, allowing for much smaller output capacitance and fast response. Finally, integrated MOSFETs and thermal management enable robust and reliable delivery of current up to 15 A continuously from input ranges up to 20 V.
About the Author
Zhongming Ye is a senior applications engineer for power products at Analog Devices in Santa Clara, California. He has been working at Linear Technology (now part of Analog Devices) since 2009 to provide application support to various products including buck, boost, flyback, and forward converters. His interests in power management include high performance power converters and regulators of high efficiency, high power density, and low EMI for automotive, medical, and industrial applications. Prior to joining Linear Technology, he worked at Intersil for three years on PWM controllers for isolated power products. He obtained a Ph.D. degree in electrical engineering from Queen’s University, Kingston, Canada. Zhongming was a senior member of the IEEE Power Electronics Society. He can be reached at [email protected].