Understanding the Real Impact of Cosmic Radiation on Power Electronics
January 03, 2025
Blog
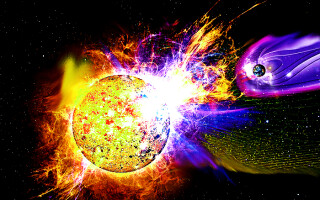
This question came up in a discussion about power electronics failures for which there was initially no viable explanation. Cosmic rays are not an invention by Stanislav Lem or a myth from the Star Wars universe. Cosmic radiation consists of high-energy particles ejected into space in large quantities by stars such as our sun. Our planet's magnetic field deflects most of what travels toward Earth, and only a few high-energy particles enter the Earth's atmosphere.
Once they enter Earth's atmosphere, they collide with other particles and pass their energy to these collision partners. As a result, more high-energy particles are produced, and further collisions create a cascade effect known as a particle shower.
In fact, this is no longer the original cosmic radiation, but the secondary radiation caused by it. Because the intensity of the radiation depends very much on the geographical altitude, it is also referred to as high-altitude radiation.
When its particles collide with the crystal structure, they still have enough energy to cause disturbances in semiconductors' electrical fields.
If the disorder propagates along the crystal lattice, a conductive channel can form in the semiconductor, and the semiconductor is destroyed. The process is shown schematically in Figure 1.
This effect is more likely to occur at high field strengths in the crystal lattice, so semiconductors currently in a blocking state are particularly affected.
|
|
|
A high-energy particle strikes the semiconductor's crystal and creates a disturbance. |
The disturbance grows around the impact site. |
A conductive tunnel forms and destroys the component. |
In the early 1990s, the influence was first noticed in power electronics. Failures that occurred in railcar traction converters could not initially be explained. A famous experiment investigated whether semiconductors in salt domes deep underground have different failure frequencies than those on mountain tops.
The result was clear: The underground systems worked without failure, while those at high altitudes failed more frequently. Because it can only be measured statistically, this failure pattern is also known as single-event burnout (SEB).
Can't you just build a shield that also works with X-rays?
As early as 1929, the German physicists Walther Bothe and Werner Kolhörster attempted to prove that the cosmic radiation postulated by Victor Franz Hess in 1912 was gamma radiation. Their experimental setup consisted of two Geiger-Müller counters and included an absorber between them, but this proved to be insufficient. Even when they used a plate of 4 cm thick gold, they found coincidences, i.e., events that occurred simultaneously in both counters. This evidence was also proof that cosmic radiation cannot be gamma radiation.
It also led to the realization that protective measures for an application or individual semiconductor assemblies against this phenomenon in an enclosure were not economically feasible.
The 1990s experiment took several kilometers of rock to provide adequate shielding.
So, what helps to keep the failure rate low, even at high altitudes?
In mathematical terms, a component's failure rate is the product of a basic failure rate that always exists, various influencing factors such as voltage and temperature, and a correction factor for geographical altitude.
High-altitude radiation itself is an uncontrollable factor. The only thing that helps is reducing the basic failure rate.
Failures in components increase with the temperature of the semiconductor. The field strength prevailing in the semiconductor is also decisive. Both can be utilized.
An oversized component in terms of current-carrying capacity has a smaller temperature swing during operation, which reduces the basic failure rate.
The ratio of the nominal blocking voltage and the voltage applied to the component can also be used. If, for example, voltages of up to 900 V are to be expected in the application, the use of 1200 V components is technically sufficient. However, using components based on 1700 V reduces the basic failure rate considerably.
How quickly does the failure rate increase with height?
Accurately measuring the failure rate as a function of the geographical height above sea level is extremely complex and has only been carried out holistically by a few institutions in the past.
The data can be used to illustrate the accelerating influence, as depicted in Figure 2.
The failure rate for power electronic components is also referred to as the FIT rate. FIT stands for Failure In Time. In industrial terms, Time is a period of 109 operating hours.
1 FIT, therefore, means that the component statistically fails within 109 hours. However, the FIT rate says nothing about when exactly the component fails or which component in a group is affected.
If a component with 100 FITs has a total of one million parts in use, a failure can be statistically assumed to occur every 10 hours.
The additional influence of cosmic radiation becomes evident in this example.
An application installed in Hamburg is 12 times more likely to fail when used in the Pilztal ski resort in Austria at 3440 meters above sea level. In La Paz, the administrative capital of Bolivia, at an altitude of 3870 meters, the probability of failure increases by a factor of 15.
This means that if a lifespan of 10 years is statistically achieved in Hamburg, this can very quickly be reduced to less than one year in the mountains.
The situation is particularly critical when using power semiconductors in aircraft or satellites.
Conclusion
High-altitude radiation as an influencing factor on the service life of a power electronic system is not a myth but a phenomenon that is now well-known and documented. Suppose failure probabilities are to be specified for general applications. In that case, it makes sense to specify their operation in electrical and thermal parameters and the geographical altitude at which the system should be used. If the developer knows in advance that the application will be used at high altitudes, suitable specifications for component selection can positively influence reliability as early as possible in the design phase.
Download the Considering Failure Rates and the Impact of Cosmic Radiation whitepaper, courtesy of Littelfuse.